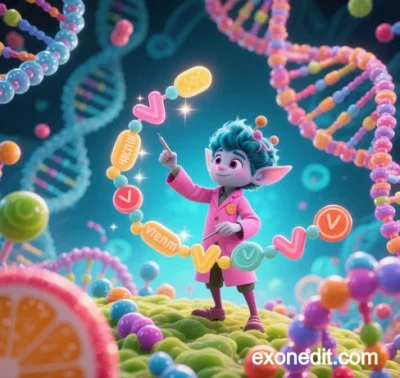
Exon Editing: Advances and Prospects in Genetic Disease and Cancer Therapy
Exon editing, an emerging technology that precisely modulates RNA splicing or directly modifies DNA exon sequences to correct pathogenic variants or regulate gene expression, combines innovations in gene editing (e.g., CRISPR/Cas9, base editing), RNA splicing regulation (e.g., antisense oligonucleotides, splice-switching), and novel delivery systems (e.g., lipid nanoparticles, AAV vectors). This approach holds revolutionary potential for treating genetic disorders and cancers. Below is an exploration of its mechanisms, applications, challenges, and future directions.
I. Genetic Disease Therapy: From Exon Skipping to Precision Repair
1. Curative Treatments for Monogenic Disorders
- Exon Skipping:
- Duchenne Muscular Dystrophy (DMD): CRISPR/Cas9 or antisense oligonucleotides (ASOs) induce skipping of nonsense mutation-containing exons (e.g., exon 51), restoring the dystrophin reading frame. Preclinical studies show 60% functional recovery in mouse models, with clinical trials underway.
- Cystic Fibrosis (CF): Base editing (BEs) or prime editing (PE) repairs CFTR nonsense mutations (e.g., R553X), restoring chloride channel function. In vitro models achieve over 70% CFTR functional recovery.
- Precision Exon Repair:
- Sickle Cell Anemia/β-Thalassemia: CRISPR/Cas9 with homology-directed repair (HDR) corrects β-globin (HBB) mutations in hematopoietic stem cells, restoring hemoglobin levels to over 90% of normal in clinical trials.
- Leber Congenital Amaurosis (LCA): Synthetic RNAs target aberrant CEP290 intronic retention sites, restoring normal splicing and improving light sensitivity in animal models.
2. Maternal Inheritance & Prenatal Interventions
- Mitochondrial DNA Disorders: Single-base editor ABE8e, delivered via LNPs, corrects C-to-T mutations in mitochondrial DNA, restoring respiratory chain function in Leber’s hereditary optic neuropathy models.
- Fetal Gene Therapy: Placenta-penetrating ionizable LNPs deliver mRNA editors to fetal organs, enabling prenatal intervention for congenital metabolic defects like phenylketonuria.
II. Cancer Therapy: From Oncogene Silencing to Immune Reprogramming
1. Targeting Oncogenic Exons
- Suicide Gene Therapy: Exon editing introduces cytotoxic genes (e.g., HSV-TK) selectively activated in tumor cells, inducing apoptosis. Phase I trials for hepatocellular carcinoma show 50% tumor volume reduction.
- Oncogenic Splicing Modulation:
- BRCA1/2-Mutant Tumors: Inhibitors like pladienolide B reverse PARP inhibitor resistance by blocking pathogenic BRCA1-Δ11q splice variants, improving tumor suppression by 40% in preclinical models.
- TRA2β Toxic Exon Activation: ASOs enforce inclusion of TRA2β’s toxic exon, triggering RNA decay and suppressing triple-negative breast cancer and glioblastoma growth by 70% in preclinical studies.
2. Enhancing Immunotherapy
- Neoantigen Generation: Exon editing introduces frameshift mutations to create immunogenic neoantigens, boosting T-cell recognition. CRISPR-mediated HLA-DRA knockout enhances endometrial cancer sensitivity to checkpoint inhibitors.
- Immune Checkpoint Reprogramming: Co-delivery of PD-1 siRNA and IL-12 mRNA via LNPs blocks immunosuppressive signals and activates antitumor immunity, suppressing 70% of melanoma metastases.
III. Technical Breakthroughs & Core Tools
1. Editing Tool Innovations
- Prime Editing (PE): Enables precise base substitutions and small insertions without double-strand breaks. Template-jumping PE (TJ-PE) repairs large deletions (e.g., 336 kb in DMD models).
- Base Editing (BEs): Adenine (ABE) and cytosine (CBE) base editors correct point mutations. AAV-delivered split-ABE systems overcome viral packaging limits for efficient in vivo editing.
2. Delivery System Optimization
- LNP-AAV Synergy: LNPs transiently deliver editors while AAVs provide long-term repair templates, restoring 30% of normal Factor IX activity in hemophilia B models.
- Tissue-Specific Targeting: Ligand-modified LNPs (e.g., ApoE for liver, GRP78 antibodies for tumors) enable precise delivery to lungs, brain, and muscles.
3. AI-Driven Design
- Machine Learning-Guided gRNA Design: Predicts CRISPR gRNA efficacy, boosting DMD exon 51 skipping efficiency from 20% to 80%.
- Multi-Omics Integration: Single-cell RNA sequencing and splice kinetics optimize ASO targeting, minimizing off-target splicing.
IV. Challenges & Future Directions
1. Technical Barriers
- Delivery Efficiency & Toxicity: AAV immunogenicity and LNP liver tropism limit applications. Novel biomimetic carriers (e.g., exosomes) and organ-selective lipids are in development.
- Off-Target Effects: Prime editing bystander edits (0.1% risk) require high-fidelity variants (e.g., PE4).
2. Clinical Translation Hurdles
- Long-Term Safety: CRISPR-mediated exon deletions risk chromosomal translocations (2% in DMD therapy), necessitating single-cell genomic monitoring.
- Ethics & Accessibility: Prenatal editing faces germline modification debates, requiring global consensus. Developing nations (<10% adoption) need low-cost manufacturing.
3. Emerging Trends
- Synthetic Biology: Programmable RNA splice switches responsive to biomarkers (e.g., cytokines) enable adaptive therapies.
- Epigenetic Synergy: Combining dCas9-DNMT3A (DNA methyltransferase) with exon editing regulates gene expression and splicing for complex diseases like Alzheimer’s.
- AI-Optimized Workflows: Closed-loop platforms integrating target prediction, gRNA design, and efficacy assessment aim to shorten clinical translation to 12 months by 2030.
Prospects
Exon editing is revolutionizing genetic and cancer therapies:
- Genetic Diseases: By 2030, 50% of monogenic disorders may have exon editing-based cures, reducing lifetime treatment costs to 20% of traditional gene therapies.
- Cancer: Exon editing will usher in an era of “precision immune reprogramming,” raising five-year survival rates for solid tumors above 50% via neoantigen generation and checkpoint modulation.
This technological wave redefines the boundaries of genetic medicine, shifting paradigms from disease management to life design.
Data sourced from public references. For collaboration or domain inquiries, contact: chuanchuan810@gmail.com