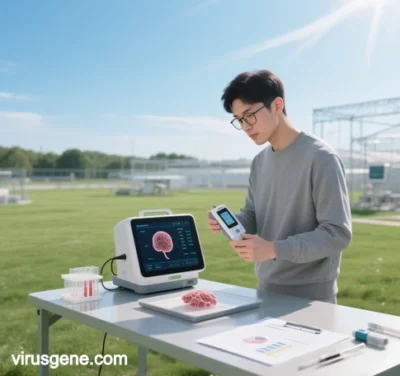
The Research Significance of Viral Genes in Medical Applications, Gene Therapy, Evolution, and Epidemiology
I. Core Roles of Viral Genes in Medical Applications
1. Breakthroughs in Gene Therapy Vectors
Genetically engineered viruses serve as efficient gene delivery tools, leveraging their natural infection mechanisms for precise therapeutic gene delivery:
- Adeno-associated viruses (AAVs): Low immunogenicity, sustained expression, and tissue specificity (e.g., retina-targeting AAV2) make them ideal for treating inherited retinal diseases and spinal muscular atrophy (e.g., Luxturna therapy).
- Lentiviruses: Enable long-term gene correction via host genome integration, used in CAR-T cell engineering and hematopoietic stem cell therapy (e.g., β-thalassemia).
- Oncolytic viruses (e.g., HSV-1): Enhanced tumor selectivity through gene editing. For example, T-VEC (Imlygic) deletes the viral ICP34.5 gene to selectively replicate in tumors and activate immune responses, approved for melanoma treatment.
2. Vaccine Development
Viral genes form the foundation of antigen delivery or live-attenuated vaccines:
- mRNA vaccines: Lipid nanoparticles (LNPs) mimic viral envelopes to deliver mRNA encoding viral proteins (e.g., Pfizer-BioNTech COVID-19 vaccine targeting spike proteins).
- Virus-like particles (VLPs): Non-infectious self-assembling particles (e.g., HPV vaccines expressing L1 proteins) combine safety and high immunogenicity.
3. Neuroscience and Regenerative Medicine
- Blood-brain barrier penetration: AAV9 modified with rabies virus glycoprotein (RVG) delivers genes for Parkinson’s and Alzheimer’s therapies.
- Neural circuit modulation: Viral vectors deliver optogenetic tools (e.g., ChR2) to specific neurons for precise neural control.
II. Challenges and Innovations in Gene Therapy
1. Balancing Vector Safety and Efficiency
- Immune evasion: Adenoviral vectors reduce immunogenicity by deleting E1/E3 genes; capsid engineering (e.g., AAV6.2FF) evades pre-existing antibodies.
- Targeted delivery: Directed evolution identifies tissue-specific capsids (e.g., AAV-PHP.eB for the central nervous system).
- Genome capacity expansion: Dual-vector systems or minimized viral elements (e.g., adenoviral ITR optimization) accommodate large genes (e.g., full-length dystrophin).
2. Delivering Gene-Editing Systems
- CRISPR-Cas9 integration: AAVs deliver SaCas9 or compact CasΦ for in vivo editing (e.g., CEP290 gene correction in inherited blindness).
- Base and epigenetic editing: Viral vectors transport ABE8e or dCas9-DNMT3A fusions for precise, cut-free epigenetic modifications.
3. Clinical Risk Management
- Insertional mutagenesis monitoring: Lentiviral self-inactivating (SIN) designs and whole-genome sequencing track clonal expansion.
- Dose toxicity control: Biodistribution studies optimize AAV dosing to reduce hepatotoxicity (e.g., avoiding liver enzyme spikes from high-dose AAV9).
III. Viral Genes in Evolution and Epidemiology
1. Decoding Viral Evolution
- Cross-species transmission: Phylogenetic analysis reveals host adaptation (e.g., SARS-CoV-2 spike RBD mutations enhancing ACE2 binding).
- Recombination hotspots: Influenza reassortment (e.g., H1N1/2009 swine-origin strain) drives pandemic risks.
2. Modeling Epidemic Dynamics
- Spatiotemporal spread networks: Whole-genome sequencing maps transmission routes (e.g., CHIKV in Cambodia) and identifies superspreader events.
- Adaptive evolution prediction: HIV-1 convergent evolution (e.g., V3 loop deglycosylation) guides vaccine design against immune escape.
3. Countering Antiviral Resistance
- Drug resistance surveillance: Global tracking of HCV NS5B inhibitor mutations (e.g., S282T) optimizes combination therapies.
- Gene drive technologies: CRISPR-engineered Wolbachia-carrying mosquitoes suppress dengue virus transmission.
IV. Interdisciplinary Integration and Future Directions
1. Synthetic Biology and Viral Engineering
- Artificial viruses: Attenuated poliovirus vectors enhance vaccine safety.
- Orthogonal systems: Engineered ribosome-codon pairs decouple viral vectors from host translation.
2. AI-Driven Viral Genome Design
- Deep learning predictions: AlphaFold2 optimizes capsid-receptor interactions for targeted delivery.
- Evolution simulation: GANs model mutation pathways to pre-identify high-risk variants.
3. Global Health Integration
- Real-time genomic surveillance: Global databases (e.g., GISAID) enable early pandemic alerts.
- Democratizing gene therapy: Modular AAV production (e.g., baculovirus-insect cell systems) lowers costs for developing regions.
Conclusion
Viral gene research bridges disease treatment and precision medicine, overcomes gene therapy bottlenecks, and provides molecular solutions to predict and combat viral threats. The integration of synthetic biology, AI, and global collaboration will usher in a “programmable therapy” era while fortifying defenses against emerging pathogens.
Data sourced from public references. Contact: chuanchuan810@gmail.com.