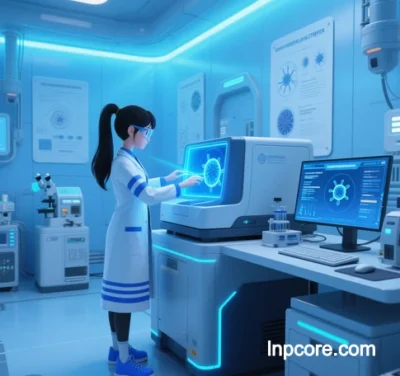
Composition and Characteristics of LNP Core (Lipid Nanoparticle Core)
The LNP (lipid nanoparticle) core is the critical delivery system for mRNA vaccines and nucleic acid therapeutics, with its composition and physicochemical properties directly determining drug stability, delivery efficiency, and targeting specificity. Below is a systematic analysis based on multidisciplinary research (2023–2025):
1. Composition of LNP Core
The LNP core comprises four key lipid components, whose molar ratios and chemical structures collectively determine performance:
- Ionizable Lipids (50–60%):
- Key Molecules: SM-102, ALC-0315, DLin-MC3-DMA.
- Function: Protonate in acidic environments (e.g., endosomes) to promote mRNA encapsulation and endosomal escape; remain electrically neutral at physiological pH to reduce cytotoxicity.
- Helper Lipids (10–15%):
- Key Molecules: DSPC, DOPE, DPPG.
- Function: Stabilize the LNP bilayer structure. DOPE enhances membrane fusion to boost cytoplasmic mRNA release.
- Cholesterol (30–40%):
- Key Molecules: Cholesterol, 20α-hydroxycholesterol.
- Function: Modulates membrane fluidity and enhances particle stability. High cholesterol content (>40%) improves mRNA encapsulation efficiency (>90%).
- PEGylated Lipids (1–5%):
- Key Molecules: PEG-DMG, ALC-0159, pSar.
- Function: Control particle size (70–150 nm) and reduce immune clearance. Excess PEG (>5%) inhibits endosomal fusion, necessitating optimization (typically <2%).
2. Key Properties and Optimization Strategies
Ionizable Lipids
- Structural Features: Comprise a headgroup (amine), linker (ester/thioester bond), and hydrophobic tail (alkyl chains).
- Headgroup Design: Piperazine (SM-102) or imidazole (ALC-0315) groups determine protonation efficiency and endosomal escape.
- Tail Design: Double-chain (C18) tails improve membrane fusion but may increase toxicity compared to single-chain (C12) tails.
- Innovations:
- Biodegradable Lipids (e.g., DLin-MC3-DMA): Ester bonds enable metabolic clearance, reducing long-term toxicity.
- pH-Responsive Lipids: Activate selectively in tumor microenvironments (pH 6.5–7.0) for enhanced targeting.
Helper Lipids
- Functional Differentiation:
- DSPC (saturated phospholipid): Increases LNP rigidity for intravenous delivery (liver targeting).
- DOPE (unsaturated phospholipid): Promotes endosomal membrane rupture, boosting mRNA release (3x higher antibody titers).
- SORT Technology: Incorporates cationic lipids (e.g., DOTAP, >20%) for extrahepatic targeting (lungs, spleen).
Cholesterol
- Dynamic Roles:
- Low Ratios (<30%): Reduce stability but minimize liver accumulation, ideal for subcutaneous/intramuscular injections.
- Functionalized Derivatives: 20α-hydroxycholesterol activates LXR pathways to enhance transfection efficiency (may trigger inflammation).
PEGylated Lipids
- Balancing Act:
- High PEG (>5%): Extends circulation half-life but suppresses endosomal escape (50% lower protein expression).
- Alternatives: Polysarcosine (pSar) reduces immunogenicity while maintaining stability.
3. Physicochemical Properties
Structural Model
- Layered Core-Shell Structure: Surface enriched with DSPC and PEGylated lipids; core contains a homogeneous mix of ionizable lipids and cholesterol.
- Phase Transition: In acidic environments, ionizable lipids protonate, shifting the core from disordered to lamellar liquid crystalline phases, facilitating mRNA release.
Size and Surface Charge
- Size Control: Microfluidics adjust particle size (70–150 nm) for organ targeting (smaller particles target liver; larger ones accumulate in spleen).
- Zeta Potential: Near 0 mV at neutral pH (minimizes nonspecific adsorption); shifts to +20–30 mV in endosomes (promotes membrane fusion).
4. Functional Characteristics and Delivery Mechanisms
Encapsulation Efficiency
- mRNA Payload: Optimal charge ratio (N/P ratio) of 6:1 (ionizable lipid to mRNA) achieves >95% encapsulation.
- Stability: Lyophilization preserves LNP activity for 24 months at 2–8°C (<10% activity loss).
Endosomal Escape
- Proton Sponge Effect: Ionizable lipids absorb protons in acidic endosomes, rupturing membranes (>80% escape efficiency).
- DOPE Synergy: Forms non-lamellar (HII) phases to destabilize endosomal membranes.
Targeting Strategies
- Passive Targeting: Liver sinusoidal endothelial cells uptake LNPs via ApoE-mediated pathways (natural liver tropism).
- Active Targeting:
- Antibody Conjugation (e.g., anti-CD3): Enhances T-cell targeting for CAR-T therapies.
- Ligand Modification (e.g., folate): Targets tumor cell receptors.
5. Clinical Challenges and Innovations
Toxicity Mitigation
- Immunogenicity: PEGylated lipids may cause allergic reactions (0.1–1% incidence), driving alternatives like pSar.
- Long-Term Safety: Metabolites of ionizable lipids (e.g., DLin-MC3-DMA LD50 >200 mg/kg) require rigorous hepatorenal toxicity evaluation.
Manufacturing Advances
- Continuous-Flow Production: Pfizer and Carbon3D’s 3D-printed microfluidic systems cut batch production from 7 days to 8 hours.
- AI-Driven Design: DeepMind’s AlphaLipid platform predicts lipid structure-performance relationships, shortening development cycles to 3 months.
6. Future Applications
Multidisease Platforms
- Cancer Vaccines: Personalized neoantigen mRNA-LNPs (e.g., targeting KRAS G12D) are in Phase II trials.
- Genetic Diseases: Inhaled LNP-mRNA restores CFTR function, showing >50% lung improvement in preclinical models.
Third-Generation LNP Technologies
- Quantum Dot Hybrids: Fluorescent tags enable real-time mRNA tracking (10 μm spatial resolution).
- Mitochondrial Targeting: Delivers mRNA to mitochondrial matrices to repair oxidative phosphorylation defects (e.g., Leigh syndrome).
Conclusion
Through innovations in ionizable lipid engineering, cholesterol modulation, and PEG alternatives, LNP cores have evolved from passive carriers to intelligent targeting systems. Modular designs and AI-driven optimization will expand mRNA therapies across oncology, genetic disorders, and infectious diseases. By 2030, the global LNP-based drug market is projected to exceed $50 billion.
Data sources: Publicly available references. For collaborations or domain inquiries, contact: chuanchuan810@gmail.com.