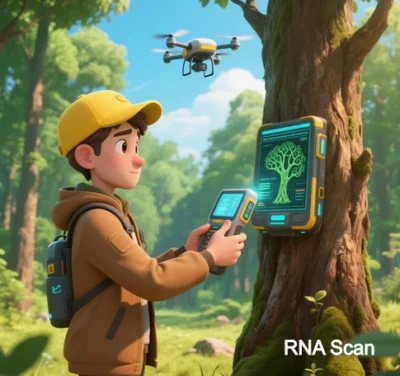
RNA Scan Technology: Core Mechanisms and Pathways for High-Precision RNA Detection
RNA Scan technology combines specific probe design and multi-level signal amplification systems to achieve highly sensitive and specific RNA detection. Below is an in-depth analysis of its core principles, technical branches, and innovative applications.
I. Specific Probe Design: The Foundation of Targeted Recognition
1. Dual Z-Probes (ZZ Probes)
- Structural Features: Each probe pair consists of two complementary “Z-shaped” oligonucleotides that bind to adjacent regions (~50 bases) on the target RNA. Signal amplification occurs only when both probes bind correctly.
- Specificity Assurance: Non-specific binding of single probes fails to form stable Z-structures, and unbound probes are washed away to prevent false positives.
- Applications: RNAscope uses 20 ZZ probe pairs to target long RNA sequences (e.g., lncRNAs ≥1 kb), enabling full-length coverage.
2. Split Initiator Probes (HiFi Probe)
- Design Logic: Probes are split into two segments, each carrying a partial initiator sequence. A complete initiator forms only when both segments bind the same RNA molecule, triggering amplification.
- Advantages: Auto-suppression of background noise enables single-molecule sensitivity (1 copy/μL).
3. Dual Competitive Padlock Probes (DCPLP)
- Innovation: Competitive binding mechanisms enhance specificity. For RNA SNP detection, non-target probes are suppressed, improving specificity by 83%.
II. Signal Amplification Systems: From Single Molecules to Visualization
1. Branched DNA (bDNA) Signal Trees
- Hierarchical Amplification:
- Pre-Amplifier: Binds probes to form a “trunk.”
- Amplifier: Attaches to pre-amplifiers, creating “branches.”
- Label Probes: Fluorescent or enzyme-labeled “leaves” complete the tree.
- Amplification Power: A fully assembled tree contains 400 labeling sites, achieving 8,000-fold amplification.
- Application: PrimeFlow RNA quantification in flow cytometry.
2. Hybridization Chain Reaction (HCR)
- Enzyme-Free Amplification: Initiator sequences trigger DNA self-assembly into fluorescently labeled long chains.
- Advantages: No protease treatment required; ideal for thick tissues (e.g., 1 cm brain slices).
3. Rolling Circle Amplification (RCA)
- Circular Probes: Padlock probes hybridize to target RNA, circularize, and extend via Phi29 polymerase to produce repetitive sequences with multiple labels.
- Dynamic Range: Detects targets as low as 10 pmol/L, suitable for clinical SNP genotyping.
III. Technical Platforms and Performance Comparison
Platform | Core Probe | Amplification Method | Sensitivity | Sample Compatibility |
---|---|---|---|---|
RNAscope | Dual Z-Probes (20 pairs) | Enzyme/fluorescence labeling | Single molecule | FFPE/frozen tissues |
ViewRNA/PrimeFlow | Targeted probe pairs (20-40) | bDNA tree amplification | 1 copy/μL | Cell suspensions, tissue sections |
HCR™ RNA-FISH | Split HiFi probes | DNA self-assembly | Single molecule | Thick tissues (≤1 cm), live cells |
DCPLP-RCA | Dual competitive padlock | Rolling circle amplification | 10 pmol/L | Clinical RNA, SNP detection |
IV. Innovative Applications
1. Tumor Microenvironment Research
- Low-Abundance RNA Detection: RNAscope locates lncRNAs (e.g., HOTAIR) in tumors to study metastasis.
- Multiplex Detection: HCR labels RNA (e.g., PD-L1 transcripts) and proteins (e.g., CD8+ T-cell markers) simultaneously to map immune checkpoint dynamics.
2. Pathogen Diagnostics
- Viral Detection: PrimeFlow + Cas13 ribozymes detect African swine fever virus in 15 minutes (1 copy/μL sensitivity).
- Drug Resistance Genotyping: DCPLP identifies CYP2C9 SNPs to guide personalized therapy.
3. Neuroscience
- Single-Neuron RNA Mapping: ViewRNA localizes neurotransmitter-related mRNAs (e.g., GAD67) in brain slices to study epilepsy.
4. Agriculture and Ecology
- Transgenic Crop Validation: RNAscope detects C4 photosynthetic gene expression in rice to evaluate yield improvements.
- Pest Control: Ribozyme probes target insect juvenile hormone genes for species-specific population regulation.
V. Challenges and Future Directions
1. Signal Stability Optimization
- Chemical Modifications: 2′-fluoro or phosphorothioate probes extend half-life to 72 hours.
- Delivery Systems: LNPs or exosomes enhance in vivo transfection efficiency (>80%).
2. AI-Driven Design Tools
- Probe Optimization: AlphaFold 3 predicts RNA-probe structures to refine binding energy.
- Quantum Computing: D-Wave annealers accelerate molecular docking simulations for probe screening.
3. High-Throughput Integration
- Microfluidic Chips: Combine probe hybridization, amplification, and detection for “sample-to-result” workflows.
- Spatial Multi-Omics: Integrate HCR with mass spectrometry imaging to map RNA, protein, and metabolite distributions.
VI. Conclusion
RNA Scan technology is driven by a dual-engine “probe-signal” framework:
- Probes ensure specificity via steric hindrance and competitive binding.
- Amplification systems (e.g., bDNA, HCR) achieve single-molecule sensitivity.
Its breakthroughs in medical diagnostics, research, and industrial applications mark the transition of RNA detection from qualitative analysis to a multi-dimensional “quantitative-spatial-dynamic” era. Future advancements in AI and synthetic biology will propel this technology toward automation and intelligence.
Data sourced from public references. Contact: chuanchuan810@gmail.com.